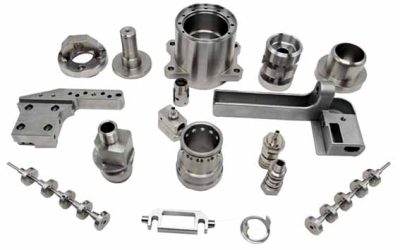
Ruostumattomasta teräksestä valmistetut osat
Kuinka koneistaa ruostumattomia osia? CNC-työstötekniikka ja ruostumattomien teräsosien käyttö
Ruostumaton teräs on monipuolinen työstömateriaali, jota voidaan käyttää laajasti paikoissa, joissa lujuus sekä lämmön- ja korroosionkestävyys ovat tärkeitä. kuitenkin, samat ominaisuudet, jotka tekevät ruostumattomista terässeoksista poikkeuksellisia rakennemateriaaleja, vaikeuttavat myös niiden koneistamiseen käytettyjä prosesseja. Leikkuutyökalun ominaisuuksien huolellinen yhdistelmä, geometriat ja leikkausparametrien soveltaminen voivat lisätä merkittävästi ruostumattoman teräksen työstöoperaatioiden tuottavuutta.
AUSTENITIC STAINLESS STEELS
They make up the 200 ja 300 series. Their general characteristics are:
Excellent corrosion resistance.
Highly formable.
Not magnetic.
Cold work hardened (Cannot be heat treated).
Excellent weldability.
They have the ability to be functional in extreme temperatures.
They are prone to corrosion cracking.
Composition:
They are obtained by adding elements such as nickel, manganese and nitrogen. The chromium content generally varies between 16 ja 26%. The carbon content is in the range of 0.03 to 0.08%. Chromium provides part of the oxidation resistance and also molybdenum in some of its alloys.
They are used in:
Shafts, valves, ruuvit, bushings, pähkinät, chemical equipment, equipment for hospitals, marine applications, aviation components, food and beverage processing equipment, architectural ornaments, cryogenic vessels, temporary prosthetics, jne.
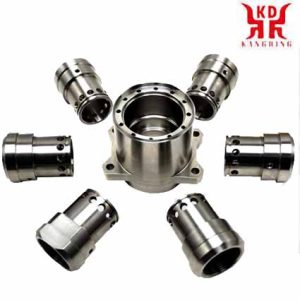
304 ruostumattomasta teräksestä valmistetut osat
Alloy evolution
Basic stainless steel alloys are classified as ferritic or martensitic. Ferritic alloys with a chromium content of 10-12 percent cannot be quenched. Martensiittisten metalliseosten kromi- ja hiilipitoisuus on korkeampi kuin ferriittisillä ruostumattomilla teräksillä, sekä mangaani- ja piipitoisuus, tuloksena on seos, joka voidaan karkaista lämpökäsittelyllä. Tänään, ferriittisiä ja martensiittisia ruostumattoman teräksen seoksia ei yleisesti käytetä teollisissa olosuhteissa, vaan pikemminkin kodin esineissä, kuten keittiö- tai puutarhatyökaluissa.
Koska ruostumattoman teräksen käyttö on kehittynyt, metalliseoksia käytetään usein tilanteissa, joissa vaaditaan mekaanista lujuutta sekä korroosionkestävyyttä. Seosten lujuuden parantamiseksi, metallurgit ovat lisänneet nikkeliä metalliseoksiin, niin se rauta / kromiseokset on muutettu raudaksi / kromi / nikkeliseokset. These materials are called austenitic stainless steels and are now common in industrial applications where strength and resistance to corrosion and heat are required. These alloys are normally used in the petrochemical industry, in the food sector, since hygiene standards require resistance to corrosion and in general machinery designed for use in harsh environments.
Inevitably, increasing the performance capabilities of an alloy, such as stainless steel, also multiplies machining problems. The corrosion resistance characteristics of martensitic and ferritic stainless steel alloys are basically chemical properties, and as a result these alloys are not much more difficult to machine than plain steels. kuitenkin, the additions of nickel and other elements to austenitic stainless steels produce higher hardness, toughness, resistance to deformation, and thermal properties that decrease machinability.
zero stainless steel 304 vs stainless steel 316
They are the most common stainless steel options. On a mechanical level they are quite similar to each other.
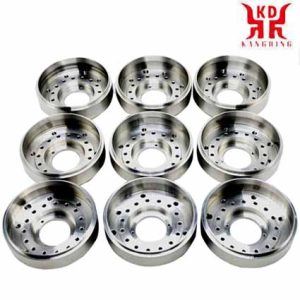
316 ruostumattomasta teräksestä valmistetut osat
316 is obtained by adding Molybdenum, which improves mechanical resistance at high temperatures compared to 304 (although its melting point is slightly lower) and resistance to corrosion against various aggressive chemicals, acids and saline atmosphere. kuitenkin, 304 is a little cheaper (important in the manufacture of large series).
Low carbon “L” types (304L and 316L) have higher corrosion resistance than basic types and reduce the possibility of corrosion cracking. They are also more suitable for welding but less mechanically resistant. The “H” tyypit, jotka osoittavat suuremman hiilen määrän, sopivat paremmin korkeisiin lämpötiloihin.
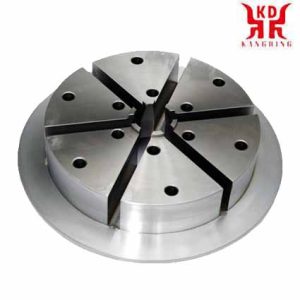
416 ruostumattomasta teräksestä valmistetut osat
MARTENSIITTISET RUOSTUMATTOMAT TERÄKSET
Ne edustavat osaa 400 series (toinen osa vastaa ferriittiä).
Sen tärkeimmät ominaisuudet ovat:
Ne voidaan kovettaa lämpökäsittelyllä, jolloin voidaan kehittää korkea mekaaninen kestävyys ja pinnan kovuus.
Niissä on suurempi mekaaninen, kulumis- ja väsymiskestävyys kuin austeniittiset.
Kohtalainen korroosionkestävyys (vähemmän kuin austeniittiset).
Hyvä koneistuskyky.
Ne ovat magneettisia.
Korkean hiilipitoisuuden ja sen kovuuden luonteen vuoksi, se on huonosti hitsattavissa.
Composition:
Ne ovat pääasiassa kromin ja hiilen seoksia. Kromipitoisuus on yleensä 10.5 to 18% ja hiilipitoisuus on korkea, saavuttavat arvot jopa 1.2%.
They are used in:
Koneiden osat, pumpun akselit, ruuvit, bushings, ruokailuvälineet, laitteisto, suihkumoottorin osat, kaivoskoneet, kiväärit, valves, aircraft accessories, inserts for fire extinguishers, parts for gas or steam turbines, rivets, jne
416 ruostumaton teräs
It is a version of type 410 (most common martensitic stainless steel), where sulfur and phosphorus are added to achieve better machining characteristics (the 400 series is already easier to machine than the 300 series). It has less ductility and formability than 410. It is more economical than the 300 series. They are for use in moderately corrosive environments. It can develop an excellent combination of mechanical resistance and hardness through adequate heat treatment.
440C stainless steel
It is possible to increase the hardness with respect to 410 by adding Carbon and the corrosion resistance is increased thanks to the chromium. Therefore they have high hardness, resistance to abrasion and wear, and good resistance to corrosion. Low machinability. This stainless steel achieves the highest hardness once hardened and the greatest resistance to wear of any steel resistant to corrosion or heat. It is widely used to make knives, bearings, measuring instruments, valve seats, jne.
PRECIPITATION HARDENED STAINLESS STEELS
This family offers an alternative to austenitic stainless steels when it is desired to combine high mechanical characteristics, good corrosion resistance and good machinability. Precipitation-hardenable steels are patented and are often designated by the initials of the manufacturing company.
Sen tärkeimmät ominaisuudet ovat:
Good corrosion resistance.
Good weldability.
Ne ovat magneettisia.
Very high mechanical strength obtained from hardening by heat aging treatment.
They maintain a high mechanical resistance even at high temperatures.
Composition:
They are mainly iron-chromium-nickel alloys. Kromipitoisuus on yleensä 12 to 18% and the nickel content is between 4% ja 9%.
They are used in:
Mainly in the aerospace and military industry (missiles) for structural components and in motor shafts, instruments, vaihteet, jne.
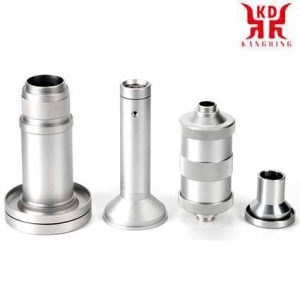
440C stainless steel parts
630 ruostumaton teräs (17-4PHE)
It offers high mechanical strength and toughness with excellent corrosion resistance. It can be hardened by aging in a simple way and at a low temperature, which avoids deformations. It is easy to machine before being heat treated.
It has a better behavior in all corrosive environments than 400 series stainless steels, and similar to 304 ruostumaton teräs. It can be welded. It may lose the high mechanical strength if heated above the precipitation hardening temperature (495-620 °C).
Know the alloys
Until recently, machining of austenitic stainless steel was not well understood. The tool manufacturers assumed that since the alloys were stronger, the mechanical cutting forces would be higher and therefore stronger negative geometry tools would have to be applied that would lower the cutting parameters. kuitenkin, this method produced tools with short life, long chips, frequent burrs, unsatisfactory surface roughness, and unwanted vibrations.
In reality, the resulting mechanical cutting forces in austenitic stainless steel are not much greater than those normally used when machining traditional steels. Most of the additional energy consumption required to machine austenitic stainless steels is the result of their thermal properties. Machining is a deformation process, and when machining a strain-resistant austenitic stainless steel, the operation generates excessive heat.
Evacuating that heat from the cutting area is vital. Unfortunately, in addition to being resistant to warping, austenitic stainless steel also has low thermal conductivity. Chips created during the machining of plain steels absorb and transport heat, but austenitic stainless steel chips absorb heat only to a limited extent. Myös, since the part itself has poor thermal conductivity, excess heat enters the cutting tool, resulting in a short tool life.
Tool manufacturers created carbide substrates to provide enough toughness to withstand the high temperatures that are generated during machining of stainless steel. Samaan aikaan, the composition of the substrate is just as important as the edge preparation. A sharper-edged tool cuts stainless steel more than warps it, thereby reducing heat generation.
Aggressive cutting parameters
In the interest of heat removal from the cutting zone, the most efficient way to machine stainless steel is to employ the greatest depths of cut and feeds. The goal is to maximize the amount of heat that is removed from the chips. Since the poor thermal conductivity of stainless steel limits the amount of heat that can be absorbed by each cubic millimeter of chip material, creating longer chips, with more cubic millimeters in volume, will remove more heat. Using greater depths of cut will also reduce the number of passes required to complete a part, an important aspect since austenitic stainless steel has a tendency to warp or harden when machined.
There are practical limitations to these aggressive machining methods. Surface finish requirements, esimerkiksi, will limit maximum feed. The available power of the machine, sekä leikkaustyökalun ja kappaleen voima, myös rajoittaa käytettävien parametrien aggressiivisuutta.
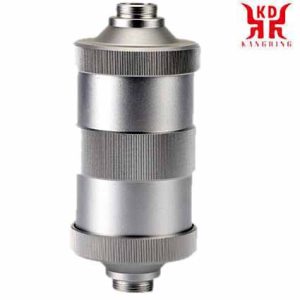
Ruostumattomien teräsosien pintakäsittely
Kylmäainestrategiat
Austeniittisten ruostumattomien terässeosten ongelmalliset lämpöominaisuudet viittaavat siihen, että jäähdytysnesteen käyttö on lähes aina kriittistä onnistuneen koneistuksen kannalta. Jäähdytysnesteen tulee olla korkealaatuista, jonka öljypitoisuus on vähintään 8–9 prosenttia vedessä / öljyemulsio, verrattuna monille koneistuksille tyypilliseen 3-4 prosentin öljypitoisuuteen.
Tärkeää on myös se, miten jäähdytysneste levitetään. Mitä suurempi paine levitettäessä jäähdytysnestettä leikkausalueelle, sitä paremmin se tekee tehtävänsä. Tekniikat, kuten Secon Jetstream Tooling®, which apply a high pressure coolant flow directly to the cutting zone, are even more effective.
Coatings vs. tool wear
A hard coating deposited on the surface of the tool substrate reinforces the hot hardness on the tool surface and improves service life in high temperature environments. kuitenkin, a coating, in general, must be thick to insulate the tool substrate from heat, and a thick coating does not adhere well to very sharp geometry. Cutting tool manufacturers are responsible for designing coatings that are thin but provide a good barrier against heat.
Austenitic stainless steels have high ductility and a tendency to stick to the cutting tool. Applying a coating can also prevent bond wear, something that occurs when machined material sticks and builds up on the cutting edge. Liimattu materiaali voi sitten rikkoa leikkuureunan osia, mikä johtaa huonoon pintakäsittelyyn ja työkaluvikaan. Pinnoite voi tarjota voitelukykyä, joka rajoittaa tikun kulumista, Suuremmat leikkausnopeudet vähentävät myös sauvan kulumismekanismia.
Jotkut austeniittiset ruostumattoman teräksen seokset sisältävät kovia ja hankaavia sulkeumia, mikä lisää leikkuutyökalun hankauskestävyyttä, yhdessä kovan pinnoitteen kanssa, voi hyötyä työkalun käyttöiästä.
Halkeilua tapahtuu metalliseosten vuoksi’ taipumus vääntyä ja itsekovettua koneistuksen aikana.
Lastua voidaan kuvata erittäin paikalliseksi äärimmäiseksi kitkakulumiseksi, ja sitä voidaan lieventää käyttämällä sopivia pinnoitteita ja muita toimenpiteitä, kuten vaihtelemalla leikkaussyvyyttä leikkuureunan kulumisvyöhykkeiden laajentamiseksi.
Työkalujen kehitys
Tool builders focus current cutting tool development on finding a balance between tool properties that will provide optimum performance on a specific material to be machined. Carbide quality research seeks a balance between hardness and toughness, so that a tool is not so hard that it fractures but is tough enough to resist deformation.
Similarly, a sharp edge geometry is preferable, although it is not as strong as a rounded edge. Siksi, the goal of developing cutting edge geometries is to create tools with a balance between sharp edge and maximum possible robustness.
As part of the development process, tool manufacturers are reviewing their guidelines on tool applications.
Current recommendations on machining parameters are based, for the most part, on the toughness and hardness of traditional steels, without taking into account the thermal factors that are so important during machining of austenitic stainless steels and other high-grade alloys. performance. Äskettäin, tool manufacturers have begun working with academic institutions to review tool testing procedures to account for the thermal characteristics of certain materials.
The new guidelines reflect the creation of new reference materials. Traditionally, machinability standards were established according to a reference material, an alloy steel, and according to the mechanical loads produced during machining. There is now a separate set of reference material for austenitic stainless steels, for which reference values have been established for speed, feed and depth of cut. With respect to the reference material, the balance or calibration factors are applied to determine the changes in the base values that will achieve optimal productivity in materials with different machining characteristics.
Specific geometries for specific materials
Many cutting tools provide very acceptable performance on various materials and under a wide variety of cutting conditions and machining parameters. For one-time jobs with moderate productivity and quality requirements, these tools can be a cost-effective option. To achieve maximum performance, kuitenkin, tool manufacturers continue to try to manipulate and balance a wide variety of cutting elements to create tools that offer high productivity and process reliability for specific materials to be machined.
The basic elements of a tool include the substrate, coating, and geometry. Jokainen niistä on tärkeä, ja parhaimmissa työkaluissa ne toimivat järjestelmänä, joka tuottaa tuloksia enemmän kuin kaikkien erillisten kappaleiden summa.
Työkalun osien toiminnoissa on eroja. Alustalla ja pinnoitteella on passiivisia toimintoja, ja ne on suunniteltu tarjoamaan tasapaino kovuuden ja sitkeyden välillä, sekä kestämään korkeita lämpötiloja, kemikaalit, tarttuvuus ja kuluminen. Työkalun geometria, toisaalta, sillä on aktiivinen rooli, koska geometriaa muuttamalla voit muuttaa tietyssä ajassa poistettavan metallin määrää, syntyvän lämmön määrä, kuinka sirut muodostuvat, ja mikä pinnan viimeistely voidaan saavuttaa.
Some basic examples of how performance changes according to different geometries include inserts with traditional Seco turning geometry called e.g. M3 and M5, which have negative cutting edge geometries (0˚ angular distance) and -T edges between the cutting edge and cutting area of the tool. The M3 geometry is a versatile semi-roughing geometry that offers good wear life and chip breakage on a wide range of machining materials. The M5 geometry is designed for demanding high feed roughing applications, combining high cutting edge strength with relatively low cutting forces.
Although versatile, M3 and M5 geometries are robust, not completely sharp-edged, and generate a fair amount of heat as they deform during machining of austenitic stainless steels. In comparison, the tool designs that can be most effective in machining stainless steel include Seco’s MF4 and MF5 geometries, which have the sharpest edge, with positive T-edges. The geometries have been designed to be open and easy to machine to facilitate medium roughing and finishing operations on steels and stainless steels. The MF5 geometry is especially effective in high feed applications.